Science Behind Auroras
By Minnie Soo 蘇慧音
Watching the light show of nature – auroras – may be on the bucket list of many people. The vibrant, magical colors were known to be mesmerizing, with green and red hues flickering and flowing gracefully like a river in the sky. This majestic natural phenomenon is particularly treasured by those of us who reside far from the poles, but have you ever wondered why do auroras mainly occur in polar regions? What is the science behind auroras? Taking a step back from the poetic imagination about auroras, you will find the formation process hardly as graceful as you might think, and underneath this beauty, lies a cascade of energetically violent events.
Solar Magnetic Field and Solar Wind
The Sun, like most stars, is a hot, fiery ball of plasma. Plasma is the fourth state of matter similar to gas, but with most of the particles ionized and moving at spectacular speeds [1]. Due to the extremely high temperature of the Sun, some particles possess sufficient kinetic energy to escape the Sun’s gravity [2]. This stream of charged ions and electrons ejected from the Sun’s surface forms what is known as the solar wind.
The flow of these fast-moving charged particles are electric currents by definition, leading to the formation of the strong, chaotic magnetic field of the Sun (Footnote 1). The solar wind also filled the solar system with a magnetic field known as the heliospheric magnetic field or interplanetary magnetic field [3]. As visualized by imaginary magnetic field lines, some of the lines form closed loops with the Sun, while others extend far out into the solar system (Figure 1) [4].
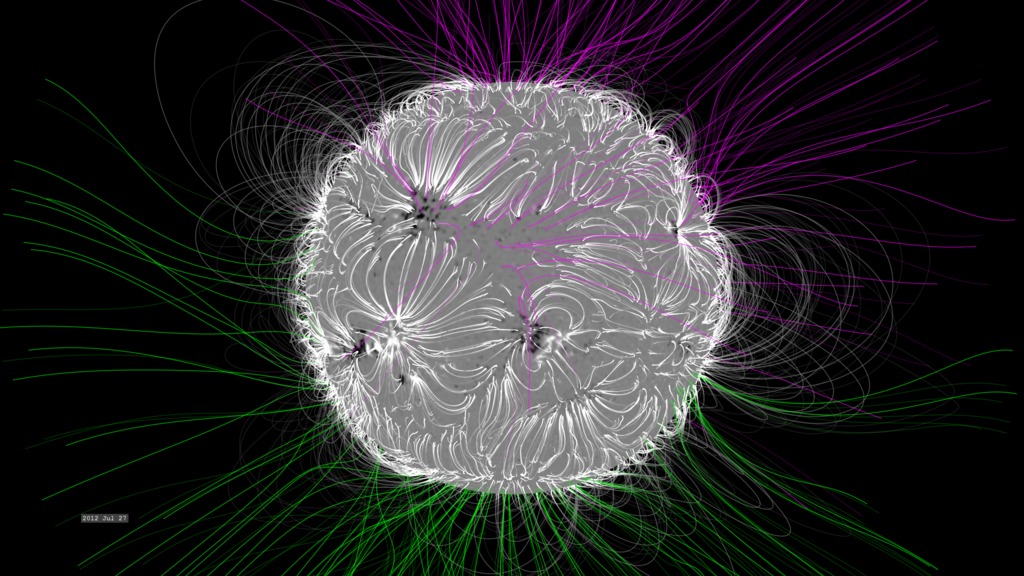
Figure 1 The solar magnetic field. White magnetic field lines are considered “closed”, while magenta and green lines are “open” field lines extending far out into the solar system with opposite magnetic polarities [4].
Photo credit: NASA's Scientific Visualization Studio
The Earth originally possesses a toroidal (donut-shaped) magnetic field. However, due to the constant bombardment of the solar wind, the magnetic field lines on the side facing the Sun was squeezed and pushed, while the magnetic field lines on the opposite side was stretched into a long, tail-like shape (Figure 2) [3]. This dynamic interaction gives Earth’s overall magnetic field a comet-like appearance. When the solar wind reaches the Earth, the charged particles are deflected by the Earth’s magnetic field, so we are shielded from the bombardment of those harmful particles [3].
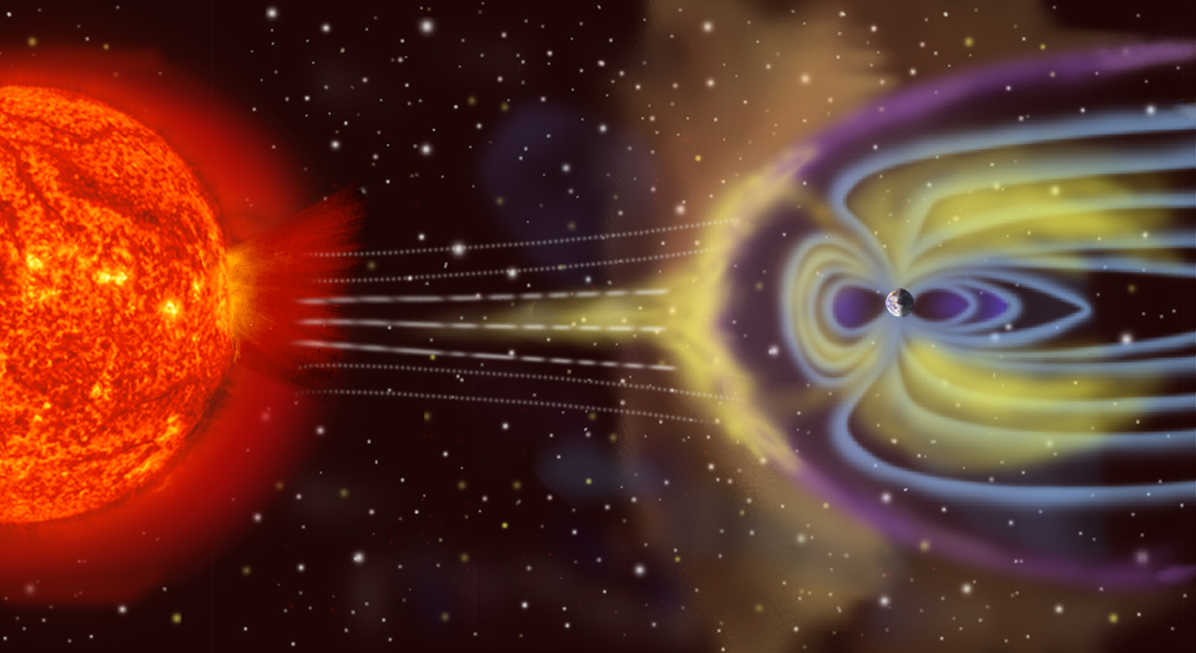
Figure 2 Artistic representation of the deflection of solar wind (greenish yellow) by the Earth’s magnetic field (blue lines). The dayside (the side facing the Sun) of the Earth’s magnetic field is compressed, while the nightside is elongated into a tail [5].
Photo credit: NASA
Magnetic Reconnection
When the Sun’s magnetic field overlaps and interacts with that of the Earth, their field lines can align in different ways [6]. Imagine pushing two magnets with the same poles against each other with tremendous force. What will happen when the oppositely pointing field lines approach each other?
Based on the idea known as “magnetic reconnection”, suggested by the Australian physicist Ronald Giovanelli in 1946, scientists discovered that misaligned magnetic fields could cause the field lines to break apart and reconnect in a whole new configuration [7, 8].
Before the reconnection occurs, as the solar wind permeated with the Sun’s magnetic field approaches the Earth, our planet’s magnetic field will be twisted, building up magnetic energy just as a stretched rubber band stores elastic potential energy. When the two fields come so close that the reconnection finally occurs, the original field lines would break up and reconnect to form a new configuration. Like suddenly letting go of the stretched rubber band, the magnetic field releases a tremendous amount of energy, accelerating the plasma particles to extremely high speeds.
Due to magnetic forces, the plasma particles travel toward the poles in helical motion along the magnetic field lines, and mirror back at the poles. They therefore bounce between the poles and are trapped in regions known as the Van Allen Radiation Belts [3, 9–11]. At the poles are two holes of the Earth’s donut-shaped magnetic field, called the polar cusps, where the charged particles will be funneled downward (Figure 3) [12]. While most particles will be mirrored back at the poles, some highly energized particles will leak out of the magnetic field through the cusps and interact with molecules in the upper atmosphere of the Earth [9].
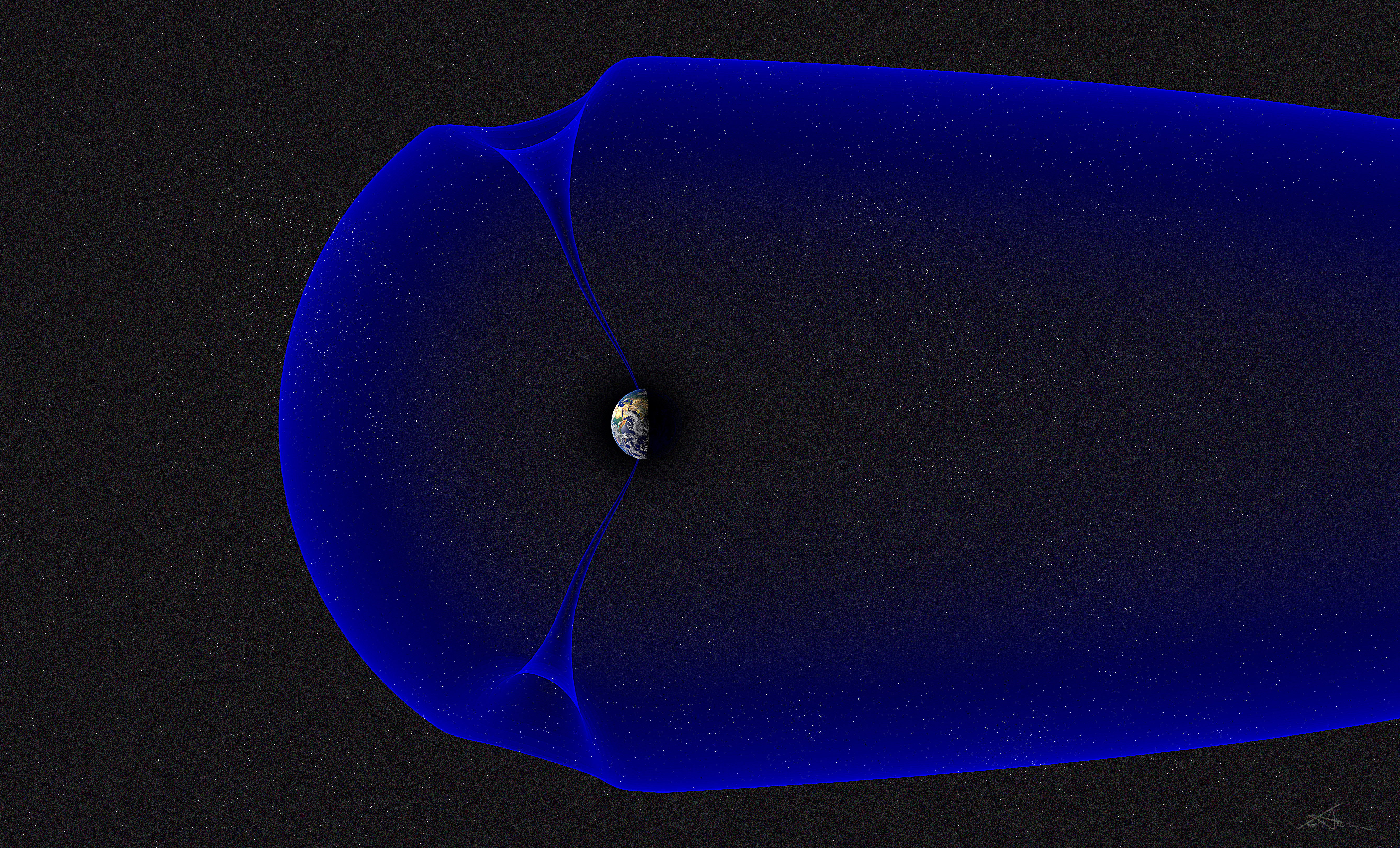
Figure 3 The Earth’s donut-shaped magnetic field with the northern and southern polar cusps where the plasma particles are funneled downward toward the poles. [13]
Photo credit: Andøya Space Center/Trond Abrahamsen
Electron Transition and Aurora Colors
The plasma particles that have arrived at the poles can then interact with the gas particles present in the second-highest layer of Earth’s atmosphere known as the thermosphere [3, 14]. While the lower part of the thermosphere is primarily composed of molecular nitrogen (N2) and molecular oxygen (O2), the upper part is dominated by monoatomic oxygen (O). Nitrogen and oxygen are crucial to the generation of auroras [3, 15].
Before delving into the mechanism, it is important to note that electrons in an atom have discrete energy levels, much like the steps on a staircase. You can walk up integer steps such as 1, 2 or 5 steps, but you cannot walk 2.3 steps or anything in between with decimal parts. Electrons can only gain certain fixed amounts of energy to be excited and jump up to specific energy levels (or release some fixed amounts of energy and return to a lower energy state), and not anywhere in between. Each atom has its own unique set of energy levels, just as fingerprints are unique to each of us.
As the plasma particles collide with the gas particles, they excite electrons within the atom. When the electron later drops back to a lower energy level, it releases energy in the form of light whose wavelength is decided by the amount of energy released. The relationship between the released energy E and the wavelength λ of emitted light is represented by the equation , where h is the constant known as Planck’s constant (6.63 × 10-34 J s) and c represents the speed of light (3.00 × 108 m s-1). We can see that the energy released is inversely proportional to the wavelength, which determines the color of light we perceive.
Atomic oxygen (O) can emit green and red lights at the wavelengths of 558 nm and 630 nm respectively [3, 16]. Molecular nitrogen (N2), on the other hand, can emit blue and red lights at multiple wavelengths which combine to form a hue of magenta at lower altitudes [3, 17]. Now you see why it matters that the energy levels are discrete: Instead of creating a continuum of all colors, only certain signature colors of auroras exist. They produce the mesmerizing display of the aurora borealis (northern lights) and aurora australis (southern lights).
1 Editor’s note: By Ampere’s law, an electric current always generates a magnetic field with a strength proportional to the current.
References
[1] Turgeon, A., & Morse, E. (2024, February 3). Sun. National Geographic Education. https://education.nationalgeographic.org/resource/sun/
[2] NASA Science. (2024). Heliophysics Big Idea 3.1. https://science.nasa.gov/learn/heat/big-ideas/big-idea-3-1/
[3] Petersen, L. (2020, September 27). What Causes the Aurora. https://www.lwpetersen.com/science-and-nature/what-causes-the-aurora/
[4] Fox, K. (2018, April 30). The Dynamic Solar Magnetic Field with Introduction. NASA's Scientific Visualization Studio. https://svs.gsfc.nasa.gov/4623/
[5] NASA. (2007, October 22). The Sun-Earth connection. The European Space Agency. https://www.esa.int/ESA_Multimedia/Images/2007/10/The_Sun-Earth_connection
[6] Frey, H. U., Han, D., Kataoka, R., Lessard, M. R., Milan, S. E., Nishimura, Y., Strangeway, R. J., & Zou, Y. (2019). Dayside Aurora. Space Science Reviews, 215. https://doi.org/10.1007/s11214-019-0617-7
[7] Day, C. (2001). Spacecraft Probes the Site of Magnetic Reconnection in Earth’s Magnetotail. Physics Today, 54(10), 16–17. https://doi.org/10.1063/1.1420541
[8] Burch, J. L., & Drake, J. F. (2009). Reconnecting Magnetic Fields. American Scientist, 97(5), 392. https://www.americanscientist.org/article/reconnecting-magnetic-fields
[9] Benesch, T. (2013, January 25). Earth’s Magnetosphere. NASA. https://www.nasa.gov/image-article/earths-magnetosphere-4/
[10] Bussio, A. (2020, May 15). Variations of particle motion in the Van Allen Belts. Journal of Research in Progress, 3. https://pressbooks.howardcc.edu/jrip3/chapter/variations-of-particle-motion-in-the-van-allen-belts/
[11] Hutchinson, I. H. (2022). Introduction to Plasma Physics. http://silas.psfc.mit.edu/introplasma/
[12] Evans, J., & Hatfield, M. (2018, November 14). Science on the Cusp: Sounding Rockets Head North. NASA. https://www.nasa.gov/solar-system/science-on-the-cusp-sounding-rockets-head-north/#hds-sidebar-nav-1
[13] NASA's Goddard Space Flight Center. (2018, September 24). Grand Challenge-Cusp Graphics. NASA Scientific Visualization Studio. https://svs.gsfc.nasa.gov/13076/
[14] The UCAR Center for Science Education. (n.d.). Auroras: The Northern and Southern Lights. https://scied.ucar.edu/learning-zone/sun-space-weather/aurora
[15] Canadian Space Agency. (2022, September 27). The colours of the northern lights. https://www.asc-csa.gc.ca/eng/astronomy/northern-lights/colours-of-northern-lights.asp
[16] National Oceanic and Atmospheric Administration. (2013, April 17). Aurora. https://sos.noaa.gov/catalog/datasets/aurora/
[17] Schmidt, T. (2024, May 14). What causes the different colours of the aurora? An expert explains the electric rainbow. UNSW Newsroom. https://www.unsw.edu.au/newsroom/news/2024/05/what-causes-the-different-colours-of-the-aurora-an-expert-explains-the-electric-rainbow